The SWI/SNF chromatin remodeling assemblies BAF and PBAF differentially regulate cell cycle exit and cellular invasion in vivo
- PMID: 34982771
- PMCID: PMC8759636
- DOI: 10.1371/journal.pgen.1009981
The SWI/SNF chromatin remodeling assemblies BAF and PBAF differentially regulate cell cycle exit and cellular invasion in vivo
Abstract
Chromatin remodelers such as the SWI/SNF complex coordinate metazoan development through broad regulation of chromatin accessibility and transcription, ensuring normal cell cycle control and cellular differentiation in a lineage-specific and temporally restricted manner. Mutations in genes encoding the structural subunits of chromatin, such as histone subunits, and chromatin regulating factors are associated with a variety of disease mechanisms including cancer metastasis, in which cancer co-opts cellular invasion programs functioning in healthy cells during development. Here we utilize Caenorhabditis elegans anchor cell (AC) invasion as an in vivo model to identify the suite of chromatin agents and chromatin regulating factors that promote cellular invasiveness. We demonstrate that the SWI/SNF ATP-dependent chromatin remodeling complex is a critical regulator of AC invasion, with pleiotropic effects on both G0 cell cycle arrest and activation of invasive machinery. Using _targeted protein degradation and enhanced RNA interference (RNAi) vectors, we show that SWI/SNF contributes to AC invasion in a dose-dependent fashion, with lower levels of activity in the AC corresponding to aberrant cell cycle entry and increased loss of invasion. Our data specifically implicate the SWI/SNF BAF assembly in the regulation of the G0 cell cycle arrest in the AC, whereas the SWI/SNF PBAF assembly promotes AC invasion via cell cycle-independent mechanisms, including attachment to the basement membrane (BM) and activation of the pro-invasive fos-1/FOS gene. Together these findings demonstrate that the SWI/SNF complex is necessary for two essential components of AC invasion: arresting cell cycle progression and remodeling the BM. The work here provides valuable single-cell mechanistic insight into how the SWI/SNF assemblies differentially contribute to cellular invasion and how SWI/SNF subunit-specific disruptions may contribute to tumorigeneses and cancer metastasis.
Conflict of interest statement
The authors have declared that no competing interests exist.
Figures
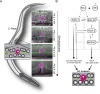
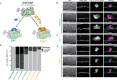
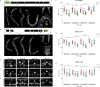
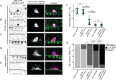
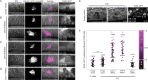
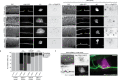
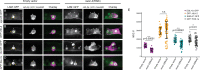
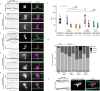
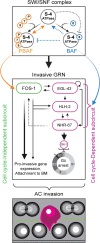
Similar articles
-
Caenorhabditis elegans SWI/SNF subunits control sequential developmental stages in the somatic gonad.G3 (Bethesda). 2014 Mar 20;4(3):471-83. doi: 10.1534/g3.113.009852. G3 (Bethesda). 2014. PMID: 24402584 Free PMC article.
-
SWI/SNF complexes act through CBP-1 histone acetyltransferase to regulate acute functional tolerance to alcohol.BMC Genomics. 2020 Sep 21;21(1):646. doi: 10.1186/s12864-020-07059-y. BMC Genomics. 2020. PMID: 32957927 Free PMC article.
-
The SWI/SNF chromatin remodeling complex exerts both negative and positive control over LET-23/EGFR-dependent vulval induction in Caenorhabditis elegans.Dev Biol. 2016 Jul 1;415(1):46-63. doi: 10.1016/j.ydbio.2016.05.009. Epub 2016 May 17. Dev Biol. 2016. PMID: 27207389
-
Opening and changing: mammalian SWI/SNF complexes in organ development and carcinogenesis.Open Biol. 2024 Oct;14(10):240039. doi: 10.1098/rsob.240039. Epub 2024 Oct 30. Open Biol. 2024. PMID: 39471843 Free PMC article. Review.
-
The SWI/SNF family of ATP-dependent chromatin remodelers: similar mechanisms for diverse functions.Curr Top Microbiol Immunol. 2003;274:143-69. doi: 10.1007/978-3-642-55747-7_6. Curr Top Microbiol Immunol. 2003. PMID: 12596907 Review.
Cited by
-
Caenorhabditis elegans for research on cancer hallmarks.Dis Model Mech. 2023 Jun 1;16(6):dmm050079. doi: 10.1242/dmm.050079. Epub 2023 Jun 6. Dis Model Mech. 2023. PMID: 37278614 Free PMC article.
-
Dynamic compartmentalization of the pro-invasive transcription factor NHR-67 reveals a role for Groucho in regulating a proliferative-invasive cellular switch in C. elegans.Elife. 2023 Dec 1;12:RP84355. doi: 10.7554/eLife.84355. Elife. 2023. PMID: 38038410 Free PMC article.
-
The Caenorhabditis elegans anchor cell transcriptome: ribosome biogenesis drives cell invasion through basement membrane.Development. 2023 May 1;150(9):dev201570. doi: 10.1242/dev.201570. Epub 2023 May 4. Development. 2023. PMID: 37039075 Free PMC article.
-
Loss of the E3 ubiquitin ligases UBR-5 or HECD-1 restores Caenorhabditis elegans development in the absence of SWI/SNF function.Proc Natl Acad Sci U S A. 2023 Jan 31;120(5):e2217992120. doi: 10.1073/pnas.2217992120. Epub 2023 Jan 23. Proc Natl Acad Sci U S A. 2023. PMID: 36689659 Free PMC article.
-
Cell cycle perturbation uncouples mitotic progression and invasive behavior in a post-mitotic cell.bioRxiv [Preprint]. 2024 Feb 7:2023.03.16.533034. doi: 10.1101/2023.03.16.533034. bioRxiv. 2024. Update in: Differentiation. 2024 May-Jun;137:100765. doi: 10.1016/j.diff.2024.100765 PMID: 38370624 Free PMC article. Updated. Preprint.
References
Publication types
MeSH terms
Substances
Grants and funding
LinkOut - more resources
Full Text Sources
Other Literature Sources