Therapeutic in vivo delivery of gene editing agents
- PMID: 35798006
- PMCID: PMC9454337
- DOI: 10.1016/j.cell.2022.03.045
Therapeutic in vivo delivery of gene editing agents
Abstract
In vivo gene editing therapies offer the potential to treat the root causes of many genetic diseases. Realizing the promise of therapeutic in vivo gene editing requires the ability to safely and efficiently deliver gene editing agents to relevant organs and tissues in vivo. Here, we review current delivery technologies that have been used to enable therapeutic in vivo gene editing, including viral vectors, lipid nanoparticles, and virus-like particles. Since no single delivery modality is likely to be appropriate for every possible application, we compare the benefits and drawbacks of each method and highlight opportunities for future improvements.
Copyright © 2022. Published by Elsevier Inc.
Conflict of interest statement
Declaration of interests The authors have filed patent applications on gene editing technologies and delivery technologies through the Broad Institute of MIT and Harvard. S.B. is a scientific cofounder and an employee of Nvelop Therapeutics. D.R.L. is a consultant and cofounder of Beam Therapeutics, Prime Medicine, Pairwise Plants, Editas Medicine, Chroma Medicine, and Nvelop Therapeutics, companies that use and/or deliver genome editing or genome engineering agents.
Figures
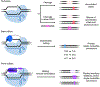
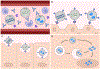
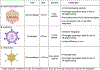
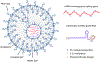
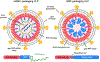
Similar articles
-
The Promise and Challenge of In Vivo Delivery for Genome Therapeutics.ACS Chem Biol. 2018 Feb 16;13(2):376-382. doi: 10.1021/acschembio.7b00680. Epub 2017 Oct 19. ACS Chem Biol. 2018. PMID: 29019396 Free PMC article. Review.
-
Delivery of CRISPR/Cas9 for therapeutic genome editing.J Gene Med. 2019 Jul;21(7):e3107. doi: 10.1002/jgm.3107. J Gene Med. 2019. PMID: 31237055 Review.
-
Delivery Aspects of CRISPR/Cas for in Vivo Genome Editing.Acc Chem Res. 2019 Jun 18;52(6):1555-1564. doi: 10.1021/acs.accounts.9b00106. Epub 2019 May 17. Acc Chem Res. 2019. PMID: 31099553 Free PMC article.
-
Viral and Non-Viral Systems to Deliver Gene Therapeutics to Clinical _targets.Int J Mol Sci. 2024 Jul 4;25(13):7333. doi: 10.3390/ijms25137333. Int J Mol Sci. 2024. PMID: 39000440 Free PMC article. Review.
-
Viral Delivery of Compact CRISPR-Cas12f for Gene Editing Applications.CRISPR J. 2024 Jun;7(3):150-155. doi: 10.1089/crispr.2024.0010. Epub 2024 May 2. CRISPR J. 2024. PMID: 38695159
Cited by
-
Enhancing cellular immunotherapies in cancer by engineering selective therapeutic resistance.Nat Rev Cancer. 2024 Sep;24(9):614-628. doi: 10.1038/s41568-024-00723-5. Epub 2024 Jul 24. Nat Rev Cancer. 2024. PMID: 39048767 Review.
-
Emerging trends in virus and virus-like particle gene therapy delivery to the brain.Mol Ther Nucleic Acids. 2024 Jul 19;35(3):102280. doi: 10.1016/j.omtn.2024.102280. eCollection 2024 Sep 10. Mol Ther Nucleic Acids. 2024. PMID: 39206077 Free PMC article. Review.
-
Assessing and advancing the safety of CRISPR-Cas tools: from DNA to RNA editing.Nat Commun. 2023 Jan 13;14(1):212. doi: 10.1038/s41467-023-35886-6. Nat Commun. 2023. PMID: 36639728 Free PMC article. Review.
-
The history, use, and challenges of therapeutic somatic cell and germline gene editing.Fertil Steril. 2023 Sep;120(3 Pt 1):528-538. doi: 10.1016/j.fertnstert.2023.02.040. Epub 2023 Mar 5. Fertil Steril. 2023. PMID: 36878350 Free PMC article. Review.
-
Advances in CAR T-cell therapy in bile duct, pancreatic, and gastric cancers.Front Immunol. 2022 Oct 6;13:1025608. doi: 10.3389/fimmu.2022.1025608. eCollection 2022. Front Immunol. 2022. PMID: 36341440 Free PMC article. Review.
References
-
- Adams D, Gonzalez-Duarte A, O'Riordan WD, Yang CC, Ueda M, Kristen AV, Tournev I, Schmidt HH, Coelho T, Berk JL, et al. (2018). Patisiran, an RNAi Therapeutic, for Hereditary Transthyretin Amyloidosis. N Engl J Med 379, 11–21. - PubMed
-
- Akinc A, Maier MA, Manoharan M, Fitzgerald K, Jayaraman M, Barros S, Ansell S, Du X, Hope MJ, Madden TD, et al. (2019). The Onpattro story and the clinical translation of nanomedicines containing nucleic acid-based drugs. Nat Nanotechnol 14, 1084–1087. - PubMed
-
- Anzalone AV, Koblan LW, and Liu DR (2020). Genome editing with CRISPR-Cas nucleases, base editors, transposases and prime editors. Nat Biotechnol 38, 824–844. - PubMed
Publication types
MeSH terms
Substances
Grants and funding
LinkOut - more resources
Full Text Sources
Other Literature Sources