Graphene oxide elicits microbiome-dependent type 2 immune responses via the aryl hydrocarbon receptor
- PMID: 36509925
- PMCID: PMC9879769
- DOI: 10.1038/s41565-022-01260-8
Graphene oxide elicits microbiome-dependent type 2 immune responses via the aryl hydrocarbon receptor
Abstract
The gut microbiome produces metabolites that interact with the aryl hydrocarbon receptor (AhR), a key regulator of immune homoeostasis in the gut1,2. Here we show that oral exposure to graphene oxide (GO) modulates the composition of the gut microbiome in adult zebrafish, with significant differences in wild-type versus ahr2-deficient animals. Furthermore, GO was found to elicit AhR-dependent induction of cyp1a and homing of lck+ cells to the gut in germ-free zebrafish larvae when combined with the short-chain fatty acid butyrate. To obtain further insights into the immune responses to GO, we used single-cell RNA sequencing to profile cells from whole germ-free embryos as well as cells enriched for lck. These studies provided evidence for the existence of innate lymphoid cell (ILC)-like cells3 in germ-free zebrafish. Moreover, GO endowed with a 'corona' of microbial butyrate triggered the induction of ILC2-like cells with attributes of regulatory cells. Taken together, this study shows that a nanomaterial can influence the crosstalk between the microbiome and immune system in an AhR-dependent manner.
© 2023. The Author(s).
Conflict of interest statement
The authors declare no competing interests.
Figures
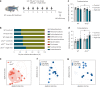
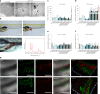
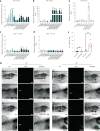
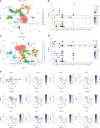
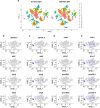
Similar articles
-
AHR2 mutant reveals functional diversity of aryl hydrocarbon receptors in zebrafish.PLoS One. 2012;7(1):e29346. doi: 10.1371/journal.pone.0029346. Epub 2012 Jan 5. PLoS One. 2012. PMID: 22242167 Free PMC article.
-
Biological effects of 6-formylindolo[3,2-b]carbazole (FICZ) in vivo are enhanced by loss of CYP1A function in an Ahr2-dependent manner.Biochem Pharmacol. 2016 Jun 15;110-111:117-29. doi: 10.1016/j.bcp.2016.04.012. Epub 2016 Apr 22. Biochem Pharmacol. 2016. PMID: 27112072 Free PMC article.
-
Zebrafish cardiotoxicity: the effects of CYP1A inhibition and AHR2 knockdown following exposure to weak aryl hydrocarbon receptor agonists.Environ Sci Pollut Res Int. 2015 Jun;22(11):8329-38. doi: 10.1007/s11356-014-3969-2. Epub 2014 Dec 23. Environ Sci Pollut Res Int. 2015. PMID: 25532870 Free PMC article.
-
Activation of aryl hydrocarbon receptor (AhR) in Alzheimer's disease: role of tryptophan metabolites generated by gut host-microbiota.J Mol Med (Berl). 2023 Mar;101(3):201-222. doi: 10.1007/s00109-023-02289-5. Epub 2023 Feb 9. J Mol Med (Berl). 2023. PMID: 36757399 Free PMC article. Review.
-
The Aryl Hydrocarbon Receptor (AHR) as a Potential _target for the Control of Intestinal Inflammation: Insights from an Immune and Bacteria Sensor Receptor.Clin Rev Allergy Immunol. 2020 Dec;59(3):382-390. doi: 10.1007/s12016-020-08789-3. Clin Rev Allergy Immunol. 2020. PMID: 32279195 Review.
Cited by
-
Environmental and Health Impacts of Graphene and Other Two-Dimensional Materials: A Graphene Flagship Perspective.ACS Nano. 2024 Feb 27;18(8):6038-6094. doi: 10.1021/acsnano.3c09699. Epub 2024 Feb 13. ACS Nano. 2024. PMID: 38350010 Free PMC article. Review.
-
The gut microbiome meets nanomaterials: exposure and interplay with graphene nanoparticles.Nanoscale Adv. 2023 Oct 18;5(23):6349-6364. doi: 10.1039/d3na00696d. eCollection 2023 Nov 21. Nanoscale Adv. 2023. PMID: 38024319 Free PMC article. Review.
-
Chiral nanoparticle-remodeled gut microbiota alleviates neurodegeneration via the gut-brain axis.Nat Aging. 2023 Nov;3(11):1415-1429. doi: 10.1038/s43587-023-00516-9. Epub 2023 Nov 9. Nat Aging. 2023. PMID: 37946041
-
Toxicological assessment of pristine and degraded forms of graphene functionalized with MnOx nanoparticles using human in vitro models representing different exposure routes.Sci Rep. 2023 Jul 22;13(1):11846. doi: 10.1038/s41598-023-38993-y. Sci Rep. 2023. PMID: 37481626 Free PMC article.
-
Vitamin D modulation of brain-gut-virome disorder caused by polystyrene nanoplastics exposure in zebrafish (Danio rerio).Microbiome. 2023 Nov 27;11(1):266. doi: 10.1186/s40168-023-01680-1. Microbiome. 2023. PMID: 38008755 Free PMC article.
References
Publication types
MeSH terms
Substances
Grants and funding
LinkOut - more resources
Full Text Sources
Miscellaneous