Electropolymerized 4-Aminobenzoic Acid Based Voltammetric Sensor for the Simultaneous Determination of Food Azo Dyes
- PMID: 36559795
- PMCID: PMC9783049
- DOI: 10.3390/polym14245429
Electropolymerized 4-Aminobenzoic Acid Based Voltammetric Sensor for the Simultaneous Determination of Food Azo Dyes
Abstract
Electrochemical sensors with polymeric films as a sensitive layer are of high interest in current electroanalysis. A voltammetric sensor based on multi-walled carbon nanotubes (MWCNTs) and electropolymerized 4-aminobenzoic acid (4-ABA) has been developed for the simultaneous determination of synthetic food azo dyes (sunset yellow FCF and tartrazine). Based on the voltammetric response of the dyes' mixture, the optimal conditions of electropolymerization have been found to be 30-fold potential scanning between -0.3 and 1.5 V, at 100 mV s-1 in the 100 µmol L-1 monomer solution in phosphate buffer pH 7.0. The poly (4-ABA)-based electrode shows a 10.5-fold increase in its effective surface area and a 17.2-fold lower electron transfer resistance compared to the glassy carbon electrode (GCE). The sensor gives a sensitive and selective response to sunset yellow FCF and tartrazine, with the peak potential separation of 232 mV in phosphate buffer pH 4.8. The electrooxidation parameters of dyes have been calculated. Simultaneous quantification is possible in the dynamic ranges of 0.010-0.75 and 0.75-5.0 µmol L-1 for both dyes, with detection limits of 2.3 and 3.0 nmol L-1 for sunset yellow FCF and tartrazine, respectively. The sensor has been tested on orange-flavored drinks and validated with chromatography.
Keywords: 4-aminobenzoic acid; electrochemical sensors; electropolymerization; food analysis; modified electrodes; sunset yellow; tartrazine.
Conflict of interest statement
The authors declare no conflict of interest.
Figures
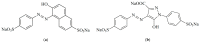
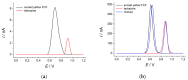
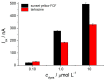
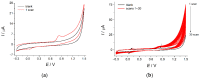
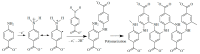
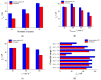
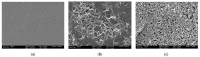
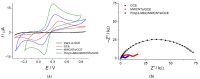
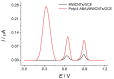
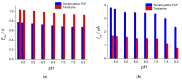
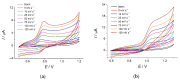
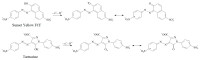
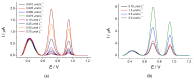
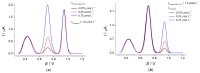
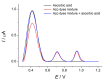
Similar articles
-
Voltammetric Sensor Based on the Combination of Tin and Cerium Dioxide Nanoparticles with Surfactants for Quantification of Sunset Yellow FCF.Sensors (Basel). 2024 Jan 31;24(3):930. doi: 10.3390/s24030930. Sensors (Basel). 2024. PMID: 38339646 Free PMC article.
-
Voltammetric Sensor Based on the Poly(p-aminobenzoic Acid) for the Simultaneous Quantification of Aromatic Aldehydes as Markers of Cognac and Brandy Quality.Sensors (Basel). 2023 Feb 20;23(4):2348. doi: 10.3390/s23042348. Sensors (Basel). 2023. PMID: 36850946 Free PMC article.
-
Selective Voltammetric Sensor for the Simultaneous Quantification of Tartrazine and Brilliant Blue FCF.Sensors (Basel). 2023 Jan 17;23(3):1094. doi: 10.3390/s23031094. Sensors (Basel). 2023. PMID: 36772133 Free PMC article.
-
Latest advances on the nanomaterials-based electrochemical analysis of azo toxic dyes Sunset Yellow and Tartrazine in food samples.Food Chem Toxicol. 2021 Oct;156:112524. doi: 10.1016/j.fct.2021.112524. Epub 2021 Aug 26. Food Chem Toxicol. 2021. PMID: 34454997 Review.
-
A comprehensive exploration of tartrazine detection in food products: Leveraging fluorescence nanomaterials and electrochemical sensors: Recent progress and future trends.Food Chem. 2024 Feb 1;433:137425. doi: 10.1016/j.foodchem.2023.137425. Epub 2023 Sep 9. Food Chem. 2024. PMID: 37690141 Review.
Cited by
-
Electrochemical Sensing Strategies for Synthetic Orange Dyes.Molecules. 2024 Oct 24;29(21):5026. doi: 10.3390/molecules29215026. Molecules. 2024. PMID: 39519667 Free PMC article. Review.
-
Voltammetric Sensor Based on the Combination of Tin and Cerium Dioxide Nanoparticles with Surfactants for Quantification of Sunset Yellow FCF.Sensors (Basel). 2024 Jan 31;24(3):930. doi: 10.3390/s24030930. Sensors (Basel). 2024. PMID: 38339646 Free PMC article.
-
Layer-by-Layer Combination of MWCNTs and Poly(ferulic acid) as Electrochemical Platform for Hesperidin Quantification.Biosensors (Basel). 2023 Apr 25;13(5):500. doi: 10.3390/bios13050500. Biosensors (Basel). 2023. PMID: 37232861 Free PMC article.
-
Voltammetric Sensor Based on the Poly(p-aminobenzoic Acid) for the Simultaneous Quantification of Aromatic Aldehydes as Markers of Cognac and Brandy Quality.Sensors (Basel). 2023 Feb 20;23(4):2348. doi: 10.3390/s23042348. Sensors (Basel). 2023. PMID: 36850946 Free PMC article.
-
Analytical Applicability of Graphene-Modified Electrode in Sunset Yellow Electrochemical Assay.Sensors (Basel). 2023 Feb 14;23(4):2160. doi: 10.3390/s23042160. Sensors (Basel). 2023. PMID: 36850755 Free PMC article.
References
Grants and funding
LinkOut - more resources
Full Text Sources