Proteomic Approaches to Uncover Salt Stress Response Mechanisms in Crops
- PMID: 36613963
- PMCID: PMC9820213
- DOI: 10.3390/ijms24010518
Proteomic Approaches to Uncover Salt Stress Response Mechanisms in Crops
Abstract
Salt stress is an unfavorable outcome of global climate change, adversely affecting crop growth and yield. It is the second-biggest abiotic factor damaging the morphological, physio-biochemical, and molecular processes during seed germination and plant development. Salt responses include modulation of hormonal biosynthesis, ionic homeostasis, the antioxidant defense system, and osmoprotectants to mitigate salt stress. Plants trigger salt-responsive genes, proteins, and metabolites to cope with the damaging effects of a high salt concentration. Enhancing salt tolerance among crop plants is direly needed for sustainable global agriculture. Novel protein markers, which are used for crop improvement against salt stress, are identified using proteomic techniques. As compared to single-technique approaches, the integration of genomic tools and exogenously applied chemicals offers great potential in addressing salt-stress-induced challenges. The interplay of salt-responsive proteins and genes is the missing key of salt tolerance. The development of salt-tolerant crop varieties can be achieved by integrated approaches encompassing proteomics, metabolomics, genomics, and genome-editing tools. In this review, the current information about the morphological, physiological, and molecular mechanisms of salt response/tolerance in crops is summarized. The significance of proteomic approaches to improve salt tolerance in various crops is highlighted, and an integrated omics approach to achieve global food security is discussed. Novel proteins that respond to salt stress are potential candidates for future breeding of salt tolerance.
Keywords: antioxidants; crops; phytohormone; proteomics; reactive oxygen species; salt stress.
Conflict of interest statement
The authors declare no conflict of interest.
Figures
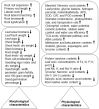
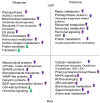
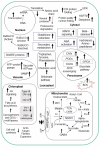
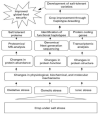
Similar articles
-
Multi-Omics Pipeline and Omics-Integration Approach to Decipher Plant's Abiotic Stress Tolerance Responses.Genes (Basel). 2023 Jun 16;14(6):1281. doi: 10.3390/genes14061281. Genes (Basel). 2023. PMID: 37372461 Free PMC article. Review.
-
Smart reprograming of plants against salinity stress using modern biotechnological tools.Crit Rev Biotechnol. 2023 Dec;43(7):1035-1062. doi: 10.1080/07388551.2022.2093695. Epub 2022 Aug 15. Crit Rev Biotechnol. 2023. PMID: 35968922 Review.
-
A comprehensive and conceptual overview of omics-based approaches for enhancing the resilience of vegetable crops against abiotic stresses.Planta. 2023 Mar 13;257(4):80. doi: 10.1007/s00425-023-04111-5. Planta. 2023. PMID: 36913037 Review.
-
TMT based proteomic profiling of Sophora alopecuroides leaves reveal flavonoid biosynthesis processes in response to salt stress.J Proteomics. 2022 Feb 20;253:104457. doi: 10.1016/j.jprot.2021.104457. Epub 2021 Dec 18. J Proteomics. 2022. PMID: 34933133
-
Multi-omics approaches for strategic improvement of stress tolerance in underutilized crop species: A climate change perspective.Adv Genet. 2019;103:1-38. doi: 10.1016/bs.adgen.2019.01.001. Epub 2019 Feb 20. Adv Genet. 2019. PMID: 30904092 Review.
Cited by
-
Effect of Shading on Physiological Attributes and Proteomic Analysis of Tea during Low Temperatures.Plants (Basel). 2023 Dec 24;13(1):63. doi: 10.3390/plants13010063. Plants (Basel). 2023. PMID: 38202371 Free PMC article.
-
Transcriptional Landscape of Cotton Roots in Response to Salt Stress at Single-cell Resolution.Plant Commun. 2023 Oct 27;5(2):100740. doi: 10.1016/j.xplc.2023.100740. Online ahead of print. Plant Commun. 2023. PMID: 39492159 Free PMC article.
References
-
- Yuvaraj M., Bose K.S.C., Elavarasi P., Tawfik E. Soil salinity and its management. In: Meena R.S., Datta R., editors. Soil Moisture Importance. IntechOpen; London, UK: 2020. - DOI
Publication types
MeSH terms
Grants and funding
LinkOut - more resources
Full Text Sources
Research Materials