Oxytocin promotes prefrontal population activity via the PVN-PFC pathway to regulate pain
- PMID: 37023755
- PMCID: PMC10272109
- DOI: 10.1016/j.neuron.2023.03.014
Oxytocin promotes prefrontal population activity via the PVN-PFC pathway to regulate pain
Abstract
Neurons in the prefrontal cortex (PFC) can provide top-down regulation of sensory-affective experiences such as pain. Bottom-up modulation of sensory coding in the PFC, however, remains poorly understood. Here, we examined how oxytocin (OT) signaling from the hypothalamus regulates nociceptive coding in the PFC. In vivo time-lapse endoscopic calcium imaging in freely behaving rats showed that OT selectively enhanced population activity in the prelimbic PFC in response to nociceptive inputs. This population response resulted from the reduction of evoked GABAergic inhibition and manifested as elevated functional connectivity involving pain-responsive neurons. Direct inputs from OT-releasing neurons in the paraventricular nucleus (PVN) of the hypothalamus are crucial to maintaining this prefrontal nociceptive response. Activation of the prelimbic PFC by OT or direct optogenetic stimulation of oxytocinergic PVN projections reduced acute and chronic pain. These results suggest that oxytocinergic signaling in the PVN-PFC circuit constitutes a key mechanism to regulate cortical sensory processing.
Keywords: PFC; PVN; hypothalamus; nociception; oxytocin; pain; paraventricular nucleus; prefrontal cortex; prelimbic.
Copyright © 2023 Elsevier Inc. All rights reserved.
Conflict of interest statement
Declaration of interests The authors declare no competing interests.
Figures
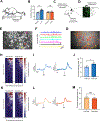
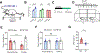
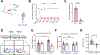
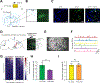
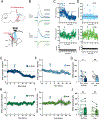
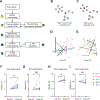
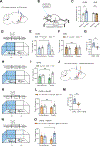
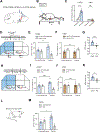
Comment in
-
Bottom-up pain regulation via oxytocin.Nat Rev Neurosci. 2023 Jun;24(6):332. doi: 10.1038/s41583-023-00708-7. Nat Rev Neurosci. 2023. PMID: 37130967 No abstract available.
Similar articles
-
Paraventricular Nucleus Oxytocin Subsystems Promote Active Paternal Behaviors in Mandarin Voles.J Neurosci. 2021 Aug 4;41(31):6699-6713. doi: 10.1523/JNEUROSCI.2864-20.2021. Epub 2021 Jul 5. J Neurosci. 2021. PMID: 34226275 Free PMC article.
-
Hypoxia activates a neuropeptidergic pathway from the paraventricular nucleus of the hypothalamus to the nucleus tractus solitarii.Am J Physiol Regul Integr Comp Physiol. 2018 Dec 1;315(6):R1167-R1182. doi: 10.1152/ajpregu.00244.2018. Epub 2018 Sep 19. Am J Physiol Regul Integr Comp Physiol. 2018. PMID: 30230933 Free PMC article.
-
The Rostral Agranular Insular Cortex, a New Site of Oxytocin to Induce Antinociception.J Neurosci. 2020 Jul 15;40(29):5669-5680. doi: 10.1523/JNEUROSCI.0962-20.2020. Epub 2020 Jun 22. J Neurosci. 2020. PMID: 32571836 Free PMC article.
-
Hypothalamospinal oxytocinergic antinociception is mediated by GABAergic and opiate neurons that reduce A-delta and C fiber primary afferent excitation of spinal cord cells.Brain Res. 2009 Jan 9;1247:38-49. doi: 10.1016/j.brainres.2008.10.030. Epub 2008 Oct 28. Brain Res. 2009. PMID: 18996098
-
_targeting Prefrontal Cortex Dysfunction in Pain.J Pharmacol Exp Ther. 2024 May 21;389(3):268-276. doi: 10.1124/jpet.123.002046. J Pharmacol Exp Ther. 2024. PMID: 38702195 Free PMC article. Review.
Cited by
-
PSD-95 Protein: A Promising Therapeutic _target in Chronic Pain.Mol Neurobiol. 2024 Sep 16. doi: 10.1007/s12035-024-04485-x. Online ahead of print. Mol Neurobiol. 2024. PMID: 39285025 Review.
-
Paraventricular Mast Cell-Derived Histamine Activates CRH Neurons to Mediate Adult Visceral Hypersensitivity Induced by Neonatal Maternal Separation.Brain Sci. 2023 Nov 16;13(11):1595. doi: 10.3390/brainsci13111595. Brain Sci. 2023. PMID: 38002554 Free PMC article.
-
PVN-mPFC OT projections modulate pup-directed pup care or attacking in virgin mandarin voles.Elife. 2024 Oct 16;13:RP96543. doi: 10.7554/eLife.96543. Elife. 2024. PMID: 39412843 Free PMC article.
-
The Role of Oxytocin in Early-Life-Stress-Related Neuropsychiatric Disorders.Int J Mol Sci. 2023 Jun 21;24(13):10430. doi: 10.3390/ijms241310430. Int J Mol Sci. 2023. PMID: 37445607 Free PMC article. Review.
-
Modulating reward and aversion: Insights into addiction from the paraventricular nucleus.CNS Neurosci Ther. 2024 Sep;30(9):e70046. doi: 10.1111/cns.70046. CNS Neurosci Ther. 2024. PMID: 39295107 Free PMC article. Review.
References
-
- Hardy SG (1985). Analgesia elicited by prefrontal stimulation. Brain Res 339, 281–284. - PubMed
Publication types
MeSH terms
Substances
Grants and funding
LinkOut - more resources
Full Text Sources
Medical
Miscellaneous