Osteocyte RANKL Drives Bone Resorption in Mouse Ligature-Induced Periodontitis
- PMID: 37551879
- PMCID: PMC11140853
- DOI: 10.1002/jbmr.4897
Osteocyte RANKL Drives Bone Resorption in Mouse Ligature-Induced Periodontitis
Abstract
Mouse ligature-induced periodontitis (LIP) has been used to study bone loss in periodontitis. However, the role of osteocytes in LIP remains unclear. Furthermore, there is no consensus on the choice of alveolar bone parameters and time points to evaluate LIP. Here, we investigated the dynamics of changes in osteoclastogenesis and bone volume (BV) loss in LIP over 14 days. Time-course analysis revealed that osteoclast induction peaked on days 3 and 5, followed by the peak of BV loss on day 7. Notably, BV was restored by day 14. The bone formation phase after the bone resorption phase was suggested to be responsible for the recovery of bone loss. Electron microscopy identified bacteria in the osteocyte lacunar space beyond the periodontal ligament (PDL) tissue. We investigated how osteocytes affect bone resorption of LIP and found that mice lacking receptor activator of NF-κB ligand (RANKL), predominantly in osteocytes, protected against bone loss in LIP, whereas recombination activating 1 (RAG1)-deficient mice failed to resist it. These results indicate that T/B cells are dispensable for osteoclast induction in LIP and that RANKL from osteocytes and mature osteoblasts regulates bone resorption by LIP. Remarkably, mice lacking the myeloid differentiation primary response gene 88 (MYD88) did not show protection against LIP-induced bone loss. Instead, osteocytic cells expressed nucleotide-binding oligomerization domain containing 1 (NOD1), and primary osteocytes induced significantly higher Rankl than primary osteoblasts when stimulated with a NOD1 agonist. Taken together, LIP induced both bone resorption and bone formation in a stage-dependent manner, suggesting that the selection of time points is critical for quantifying bone loss in mouse LIP. Pathogenetically, the current study suggests that bacterial activation of osteocytes via NOD1 is involved in the mechanism of osteoclastogenesis in LIP. The NOD1-RANKL axis in osteocytes may be a therapeutic _target for bone resorption in periodontitis. © 2023 The Authors. Journal of Bone and Mineral Research published by Wiley Periodicals LLC on behalf of American Society for Bone and Mineral Research (ASBMR).
Keywords: LIGATURE-INDUCED PERIODONTITIS; NOD1; OSTEOCLASTS; OSTEOCYTES; RANKL.
© 2023 The Authors. Journal of Bone and Mineral Research published by Wiley Periodicals LLC on behalf of American Society for Bone and Mineral Research (ASBMR).
Figures
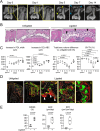
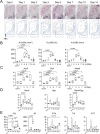
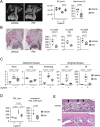
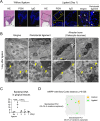
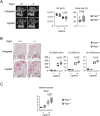
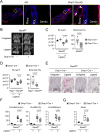
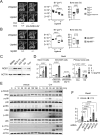
Similar articles
-
Inhibition of osteocyte apoptosis prevents the increase in osteocytic receptor activator of nuclear factor κB ligand (RANKL) but does not stop bone resorption or the loss of bone induced by unloading.J Biol Chem. 2015 Jul 31;290(31):18934-42. doi: 10.1074/jbc.M115.642090. Epub 2015 Jun 17. J Biol Chem. 2015. PMID: 26085098 Free PMC article.
-
Osteocyte-Related Cytokines Regulate Osteoclast Formation and Bone Resorption.Int J Mol Sci. 2020 Jul 21;21(14):5169. doi: 10.3390/ijms21145169. Int J Mol Sci. 2020. PMID: 32708317 Free PMC article. Review.
-
Osteocytes play an important role in experimental periodontitis in healthy and diabetic mice through expression of RANKL.J Clin Periodontol. 2018 Mar;45(3):285-292. doi: 10.1111/jcpe.12851. Epub 2018 Jan 25. J Clin Periodontol. 2018. PMID: 29220094 Free PMC article.
-
DC-STAMP Is an Osteoclast Fusogen Engaged in Periodontal Bone Resorption.J Dent Res. 2017 Jun;96(6):685-693. doi: 10.1177/0022034517690490. Epub 2017 Feb 15. J Dent Res. 2017. PMID: 28199142 Free PMC article.
-
The Osteocyte as a Novel Key Player in Understanding Periodontitis Through its Expression of RANKL and Sclerostin: a Review.Curr Osteoporos Rep. 2019 Jun;17(3):116-121. doi: 10.1007/s11914-019-00509-x. Curr Osteoporos Rep. 2019. PMID: 30924022 Free PMC article. Review.
Cited by
-
Unraveling ferroptosis in osteogenic lineages: implications for dysregulated bone remodeling during periodontitis progression.Cell Death Discov. 2024 Apr 26;10(1):195. doi: 10.1038/s41420-024-01969-6. Cell Death Discov. 2024. PMID: 38670955 Free PMC article.
-
Type VI Collagen Deficiency Causes Enhanced Periodontal Tissue Destruction.J Dent Res. 2024 Aug;103(9):878-888. doi: 10.1177/00220345241256306. Epub 2024 Jun 24. J Dent Res. 2024. PMID: 38910439
-
Loss-of-function OGFRL1 variants identified in autosomal recessive cherubism families.JBMR Plus. 2024 Apr 9;8(6):ziae050. doi: 10.1093/jbmrpl/ziae050. eCollection 2024 Jun. JBMR Plus. 2024. PMID: 38699440 Free PMC article.
-
Inhibition of RANKL improves the skeletal phenotype of adenine-induced chronic kidney disease in mice.JBMR Plus. 2024 Jan 14;8(2):ziae004. doi: 10.1093/jbmrpl/ziae004. eCollection 2024 Feb. JBMR Plus. 2024. PMID: 38505524 Free PMC article.
References
-
- Phipps KR, Stevens VJ.. Relative contribution of caries and periodontal disease in adult tooth loss for an HMO dental population. J Public Health Dent. 1995;55(4):250–252. - PubMed