Lipid Deficiency Contributes to Impaired Alveolar Progenitor Cell Function in Aging and Idiopathic Pulmonary Fibrosis
- PMID: 38657143
- PMCID: PMC11299087
- DOI: 10.1165/rcmb.2023-0290OC
Lipid Deficiency Contributes to Impaired Alveolar Progenitor Cell Function in Aging and Idiopathic Pulmonary Fibrosis
Abstract
Idiopathic pulmonary fibrosis (IPF) is an aging-associated interstitial lung disease resulting from repeated epithelial injury and inadequate epithelial repair. Alveolar type II cells (AEC2s) are progenitor cells that maintain epithelial homeostasis and repair the lung after injury. In the current study, we assessed lipid metabolism in AEC2s from human lungs of patients with IPF and healthy donors, as well as AEC2s from bleomycin-injured young and old mice. Through single-cell RNA sequencing, we observed that lipid metabolism-related genes were downregulated in IPF AEC2s and bleomycin-injured mouse AEC2s. Aging aggravated this decrease and hindered recovery of lipid metabolism gene expression in AEC2s after bleomycin injury. Pathway analyses revealed downregulation of genes related to lipid biosynthesis and fatty acid β-oxidation in AEC2s from IPF lungs and bleomycin-injured, old mouse lungs compared with the respective controls. We confirmed decreased cellular lipid content in AEC2s from IPF lungs and bleomycin-injured, old mouse lungs using immunofluorescence staining and flow cytometry. Futhermore, we show that lipid metabolism was associated with AEC2 progenitor function. Lipid supplementation and PPARγ (peroxisome proliferator activated receptor γ) activation promoted progenitor renewal capacity of both human and mouse AEC2s in three-dimensional organoid cultures. Lipid supplementation also increased AEC2 proliferation and expression of SFTPC in AEC2s. In summary, we identified a lipid metabolism deficiency in AEC2s from lungs of patients with IPF and bleomycin-injured old mice. Restoration of lipid metabolism homeostasis in AEC2s might promote AEC2 progenitor function and offer new opportunities for therapeutic approaches to IPF.
Keywords: aging; alveolar progenitor cells; idiopathic pulmonary fibrosis; lipid metabolism; three-dimensional organoid culture.
Figures
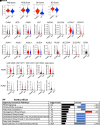
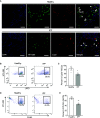
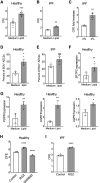
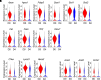
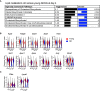
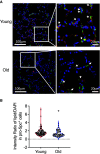
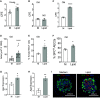
Comment in
-
Lipids to the Rescue in Pulmonary Fibrosis: Biosynthesis, Bioenergetics, or Epigenetics?Am J Respir Cell Mol Biol. 2024 Aug;71(2):143-145. doi: 10.1165/rcmb.2024-0187ED. Am J Respir Cell Mol Biol. 2024. PMID: 38713058 Free PMC article. No abstract available.
Similar articles
-
Reciprocal interactions between alveolar progenitor dysfunction and aging promote lung fibrosis.Elife. 2023 Jun 14;12:e85415. doi: 10.7554/eLife.85415. Elife. 2023. PMID: 37314162 Free PMC article.
-
The ZIP8/SIRT1 axis regulates alveolar progenitor cell renewal in aging and idiopathic pulmonary fibrosis.J Clin Invest. 2022 Jun 1;132(11):e157338. doi: 10.1172/JCI157338. J Clin Invest. 2022. PMID: 35389887 Free PMC article.
-
DNA repair enzyme OGG1 promotes alveolar progenitor cell renewal and relieves PM2.5-induced lung injury and fibrosis.Ecotoxicol Environ Saf. 2020 Dec 1;205:111283. doi: 10.1016/j.ecoenv.2020.111283. Epub 2020 Sep 22. Ecotoxicol Environ Saf. 2020. PMID: 32977282
-
Senescence of alveolar epithelial progenitor cells: a critical driver of lung fibrosis.Am J Physiol Cell Physiol. 2023 Aug 1;325(2):C483-C495. doi: 10.1152/ajpcell.00239.2023. Epub 2023 Jul 17. Am J Physiol Cell Physiol. 2023. PMID: 37458437 Free PMC article. Review.
-
_targeting Alveolar Repair in Idiopathic Pulmonary Fibrosis.Am J Respir Cell Mol Biol. 2021 Oct;65(4):347-365. doi: 10.1165/rcmb.2020-0476TR. Am J Respir Cell Mol Biol. 2021. PMID: 34129811 Free PMC article. Review.
Cited by
-
Lipids to the Rescue in Pulmonary Fibrosis: Biosynthesis, Bioenergetics, or Epigenetics?Am J Respir Cell Mol Biol. 2024 Aug;71(2):143-145. doi: 10.1165/rcmb.2024-0187ED. Am J Respir Cell Mol Biol. 2024. PMID: 38713058 Free PMC article. No abstract available.
-
Aging-Associated Molecular Changes in Human Alveolar Type I Cells.J Respir Biol Transl Med. 2024 Sep;1(3):10012. doi: 10.35534/jrbtm.2024.10012. Epub 2024 Jul 22. J Respir Biol Transl Med. 2024. PMID: 39220636 Free PMC article.
References
MeSH terms
Substances
Grants and funding
LinkOut - more resources
Full Text Sources
Medical