A biomimetic approach to modulating the sustained release of fibroblast growth factor 2 from fibrin microthread scaffolds
- PMID: 39070763
- PMCID: PMC11274095
- DOI: 10.37349/ebmx.2024.00006
A biomimetic approach to modulating the sustained release of fibroblast growth factor 2 from fibrin microthread scaffolds
Abstract
Aim: The pleiotropic effect of fibroblast growth factor 2 (FGF2) on promoting myogenesis, angiogenesis, and innervation makes it an ideal growth factor for treating volumetric muscle loss (VML) injuries. While an initial delivery of FGF2 has demonstrated enhanced regenerative potential, the sustained delivery of FGF2 from scaffolds with robust structural properties as well as biophysical and biochemical signaling cues has yet to be explored for treating VML. The goal of this study is to develop an instructive fibrin microthread scaffold with intrinsic topographic alignment cues as well as regenerative signaling cues and a physiologically relevant, sustained release of FGF2 to direct myogenesis and ultimately enhance functional muscle regeneration.
Methods: Heparin was passively adsorbed or carbodiimide-conjugated to microthreads, creating a biomimetic binding strategy, mimicking FGF2 sequestration in the extracellular matrix (ECM). It was also evaluated whether FGF2 incorporated into fibrin microthreads would yield sustained release. It was hypothesized that heparin-conjugated and co-incorporated (co-inc) fibrin microthreads would facilitate sustained release of FGF2 from the scaffold and enhance in vitro myoblast proliferation and outgrowth.
Results: Toluidine blue staining and Fourier transform infrared spectroscopy confirmed that carbodiimide-conjugated heparin bound to fibrin microthreads in a dose-dependent manner. Release kinetics revealed that heparin-conjugated fibrin microthreads exhibited sustained release of FGF2 over a period of one week. An in vitro assay demonstrated that FGF2 released from microthreads remained bioactive, stimulating myoblast proliferation over four days. Finally, a cellular outgrowth assay suggests that FGF2 promotes increased outgrowth onto microthreads.
Conclusions: It was anticipated that the combined effects of fibrin microthread structural properties, topographic alignment cues, and FGF2 release profiles will facilitate the fabrication of a biomimetic scaffold that enhances the regeneration of functional muscle tissue for the treatment of VML injuries.
Keywords: Fibroblast growth factor 2; fibrin; fibrin microthreads; myoblast; skeletal muscle; tissue engineering.
Conflict of interest statement
Conflicts of interest The authors declare that they have no conflicts of interest.
Figures
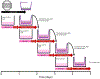

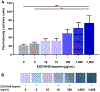
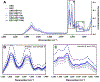
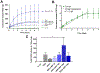
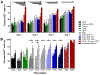
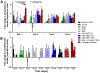
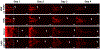
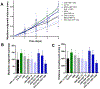
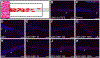
Similar articles
-
* Design of an In Vitro Model of Cell Recruitment for Skeletal Muscle Regeneration Using Hepatocyte Growth Factor-Loaded Fibrin Microthreads.Tissue Eng Part A. 2017 Aug;23(15-16):773-783. doi: 10.1089/ten.TEA.2016.0440. Epub 2017 May 18. Tissue Eng Part A. 2017. PMID: 28351217 Free PMC article.
-
Etching anisotropic surface topography onto fibrin microthread scaffolds for guiding myoblast alignment.J Biomed Mater Res B Appl Biomater. 2020 Jul;108(5):2308-2319. doi: 10.1002/jbm.b.34566. Epub 2020 Jan 22. J Biomed Mater Res B Appl Biomater. 2020. PMID: 31967415 Free PMC article.
-
Horseradish Peroxidase-Catalyzed Crosslinking of Fibrin Microthread Scaffolds.Tissue Eng Part C Methods. 2020 Jun;26(6):317-331. doi: 10.1089/ten.TEC.2020.0083. Epub 2020 Jun 9. Tissue Eng Part C Methods. 2020. PMID: 32364015 Free PMC article.
-
Biomimetic scaffolds for regeneration of volumetric muscle loss in skeletal muscle injuries.Acta Biomater. 2015 Oct;25:2-15. doi: 10.1016/j.actbio.2015.07.038. Epub 2015 Jul 26. Acta Biomater. 2015. PMID: 26219862 Free PMC article. Review.
-
Skeletal Muscle Tissue Engineering: Biomaterials-Based Strategies for the Treatment of Volumetric Muscle Loss.Bioengineering (Basel). 2020 Jul 31;7(3):85. doi: 10.3390/bioengineering7030085. Bioengineering (Basel). 2020. PMID: 32751847 Free PMC article. Review.
Cited by
-
Hydrogels in cardiac tissue engineering: application and challenges.Mol Cell Biochem. 2024 Nov 4. doi: 10.1007/s11010-024-05145-3. Online ahead of print. Mol Cell Biochem. 2024. PMID: 39495368 Review.
References
-
- National hospital discharge survey [Internet]. Atlanta: Center for Disease Control; c2010. [cited 2020 Aug 5]. Available from: https://www.cdc.gov/nchs/nhds/index.htm
-
- Ambulatory health care data [Internet]. Atlanta: Center for Disease Control; c2010. [cited 2020 Aug 5]. Available from: https://www.cdc.gov/nchs/ahcd/index.htm
-
- National hospital ambulatory medical care survey: 2021 emergency department summary tables [Internet]. Atlanta: Center for Disease Control; c2010. [cited 2020 Aug 5]. Available from: https://www.cdc.gov/nchs/data/nhamcs/web_tables/2021-nhamcs-ed-web-table...
-
- List of publications using data from NAMCS and NHAMCS [Internet]. Atlanta: Center for Disease Control; c2010. [cited 2020 Aug 5]. Available from: https://www.cdc.gov/nchs/data/ahcd/namcs_nhamcs_publication_list.pdf
-
- Medical Expenditures Panel Survey (MEPS) [Internet]. Rockville: Agency for Healthcare Research and Quality; [cited 2020 Aug 5]. Available from: https://meps.ahrq.gov/mepsweb/
Grants and funding
LinkOut - more resources
Full Text Sources